It is no coincidence that most of the clinically important multidrug resistant bacteria can persist in humans as harmless commensals, as well as being able to cause disease.
Commensals, by their nature, can persist in long term infections and are therefore subject to ‘collateral’ exposure to antibiotics when they are not the target of therapy. Commensal microbes can therefore accumulate antibiotic resistance traits and then pose risks to their human hosts when we undergo medical interventions such as surgery, chemotherapy or even simply catheterization. Thorough meta-analyses studies have shown how completing a course of antibiotics leads to an elevated risk of drug-resistant infections for 6-12 months – a risk almost certainly derived from commensal infection. Carriage of drug-resistant commensal microbes is therefore potentially serious for individuals, aside from the general problems of transmission of antibiotic resistant bacteria to the wider population.
In fact, for certain operations – organ transplants and abdominal surgery in particular – colonization by Gram-negative drug resistant bacteria is particularly risky and may prevent surgery taking place. This has led to the development of ‘decolonization’ strategies that are designed to remove persistent drug resistant commensal infections from organs such as the intestine.
The problem with established decolonization approaches is that they rely on the use of even more antimicrobials, a flawed solution to the problem of antimicrobial resistance.
Other softer biological approaches have been attempted and these typically rely on either competition from probiotics or the use of phage. However, biological methods can also have their flaws. Probiotics commonly have weak or variable clinical impacts. From an ecological perspective, probiotics have an uphill struggle as tools for decolonization. The target we are attempting to displace is likely to be more numerous and many also be at a physiological advantage by being safely embedded in a biofilm or the mucosa lining the intestine. Numerical disadvantages are particularly important if we are relying on the production of antimicrobial peptides (bacteriocins) by probiotics as a mechanism of displacement. These peptides are believed to be important for the efficacy some well-known probiotics such as the Nissle strain of Escherichia coli. Nevertheless, ecological studies have shown that bacteriocin mediated competition typically follows a pattern of frequency dependence: more numerous strains will have higher concentrations of peptides to target against the opposition and will in turn endure lower concentrations of any bacteriocins produced by the competition. These frequency dependent interactions are predicted to produce quite stable infections that may create a ‘winner stays on’ scenario in which it is hard to remove residents.
Phages clearly have great potential in reducing the density of targets and their increasingly widespread use in compassionate therapy is re-building their reputation. Nevertheless, they are typically susceptible to the evolution of resistance. Phages are therefore predominantly used in cocktails or sometimes in combination with antibiotics to improve efficacy and reduce the fitness of mutants resistant to a single phage. Our strategy for decolonization was to test if combining phage predation and probiotic competition would be particularly effective. There was a clear ecological rationale for this combination: phage works most effectively at a high density of targets and can bring down population sizes very sharply. When a decolonization target is at low density, we would it expect to be more susceptible to competitive interactions from other bacteria because of the frequency dependent fitness effects described above. In addition, there is evidence that the fitness costs of resistance can be elevated when bacteria are competing or otherwise under stress - so there is the possibility that any phage-resistant genotypes that arise after the use of phage will be even easier to displace than their susceptible counterparts.
Our study used the multidrug resistant epidemic clone Escherichia coli ST131 as a target. This strain is extremely important globally as a cause of hospital-acquired infections, sepsis and urinary tract infections. It also presents a relatively narrow genetic target for phages which typically have narrow host range. The risks from colonization of ST131 are also well understood, catherization provides a transmission route for the microbe that allows it to move from the intestinal tract, to the bladder and thereafter to the kidney and bloodstream in more serious infections. Although our research was carried out in vitro and is therefore “proof of principle”, the results were very encouraging. Across multiple experiments, and in both anaerobic and aerobic conditions, we found that combinations of phage and probiotic Nissle produced multiple order of magnitude benefits in terms of reducing the density of targets. When we used Nissle alone it showed the frequency dependent efficacy typical of bacteriocin mediated competition: it could only displace E. coli ST131 when the probiotic was at high frequency (90% or more in a mixed culture). As expected, the use of single phage alone can reduce the density of a target - but this effect only lasts for one or two transfers: resistance soon evolves to fixation and bacterial densities bounce back. In contrast, combination of phage and probiotic could break the frequency dependent advantage of an ST131 competitor, even when this target was at high frequency. While we did not manage to drive ST131 to extinction we did reduce it to a new low stable frequency. This low frequency persisted for more than 10 broth transfers – over 100 generations.
Ours is not the only study to have shown the potential of phage-probiotic combinations. While we were preparing our article for submission, a similar study targeting drug resistant E. coli associated with pigs was published. There are differences in these studies in that the Australian team managed to eliminate their target to below a detection threshold, while we switched ST131 to a dynamic state in which it persisted at a new, much lower frequency. The reasons for these different dynamics are not clear; both studies identified a phage with specific mode of action that would not infect the probiotic; both studies used competitors with good bacteriocin production. Currently we are intrigued by the possibility that phage resistance in E.coli ST131 has led to increased susceptibility to the microcins secreted by E. coli Nissle. This could explain some of the patterns we saw but as yet requires further work.
This research raises many questions as well as a number of possibilities. Ideally, in future we would prefer to work with a phage cocktail that mitigates against the evolution of resistance to phage. Translating this research to human or other vertebrate hosts will present a number of challenges. The intestine is a challenging target for phage therapy. Bacteria are likely to be in many spatial foci of infection and may be physically protected from attack by phage. Nevertheless, progress is being made in terms of understanding how to improve how phage bind to bacteria in cryptic niches such as the gut.
The current regulatory climate, both in the UK and the EU makes it challenging to carry out clinical trials with phage.
Nevertheless, as the number of successful compassionate use cases climbs, we can be optimistic that phage therapy will become a better understood and more acceptable clinical intervention.
Our suggested combination of phage and probiotic has several potential benefits. E. coli Nissle has been widely available for sale in a number of countries for decades and has a long history of safe use. This targeted use of phage exploits some of the particular biology of these viruses. In typical curative clinical applications aimed at life threatening infections, the narrow host range of phage can be problematic as there is a race against time to identify and source an effective phage against a particular causative agent. ST131 is such a widespread problem that the target is known in advance - although not every isolate within this recent clonal expansion will have identical patterns of susceptibility to phage. Nevertheless, a relatively narrow host range is an advantage – it will hopefully allow us to target one particular genotype and leave the rest of the intestinal community intact.
Currently one of the frustrating aspects of the problem of the rise of antimicrobial resistance is that we have so few tools to deploy. We can certainly reduce prescribing rates and limit the more widespread use of antibiotics. We can also alter the pattern of prescribing in time and space (although the benefits of that are intensely debated). New drugs are often lauded as a solution, but with business-as-usual prescribing they might only constitute a delaying tactic. The active removal of dangerous drug resistant infections is a very valuable approach that has already proved its worth in the context of the management of methicillin resistant Staphylococcus aureus (MRSA). Gram-negative microbes are proving more challenging targets for decolonization but the prevalence and importance of the super bug E. coli ST131 means that we have at least one well-defined target to aim at – a target that can be used in the search for alternative therapeutic agents such as phage.
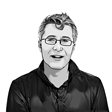
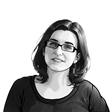
No comments yet