Imagining what life is like for microorganisms in the ground beneath our feet is difficult. Perhaps we can visualise how soils carry a wide variety of microorganisms in an environment where there is plenty of space between soil particles and where water flows freely supplying the microbes with nutrients and energy, but what happens as we go deeper?
As soil gives way to rock, as nutrients and energy sources coming from the surface get used up, as the spaces for water flow decrease and temperature increase, what happens to the microbial communities present?
These might not seem like the kind of environments that encourages huge amounts of microbial activity and, on the whole, you’d be right. In fact, the deep subsurface was thought to be sterile (which seems to be a result of assuming that the findings at one site with especially low microbial levels could be applied everywhere). However, we now know that is not the case and that microbes exist several kilometres beneath the subsurface until it becomes too hot, too salty or otherwise inhospitable for microbes. Typically, life in the deep proceeds at a sedate pace limited by the supply of nutrients amongst other things. However, the sheer volume occupied by this habitat means that even at low levels of activity, microorganisms can be important contributors to global nutrient cycles. In some cases, these types of environments are also important for understanding what early life might have looked like on Earth and can provide insights into the potential for life on other planets.
Fascinating though it is to probe questions on those topics, it is not the focus of the work in our laboratory at the British Geological Survey. Instead, we focus on what happens when these environments become disturbed by human activity. In particular, we concentrate on the effects on industries that are part of our efforts to decarbonise our energy supply and make efficient use of resources. At first glance, the role which microorganisms play in this might not be obvious, but any intrusion into underground environments disturbs them in ways that affect the microbial communities present and may introduce new microorganisms to that environment.
The general question we seek to answer is can the activity of these disturbed microbial communities affect industrial activities, for good or ill?
Take, for example, the disposal of radioactive waste. Whether we like it or not, we have a legacy of radioactive waste material that has been used in processes such as energy generation and medical and scientific research, and this waste needs to be disposed of safely. Disposal in a specially designed repository, constructed deep below the surface in suitable geological setting, is considered to be the safest option. Before this is done we need to have assurance that this process will be safe, and need to explore all possible scenarios, including the potential for microbial impacts. As you excavate this repository, you will open up space, affect the flow of water and introduce oxygen into what was previously the realm of anaerobic microorganisms. The damage caused by excavation might allow microorganisms to grow within, in rocks that previously had pore spaces too small for microorganisms to fit. As you construct your repository you will change the environment in other ways and introduce materials that remain after the repository has been filled. After the repository is closed, a complex series of changes to the environmental conditions will take place, eventually returning the site to anoxic conditions. The questions we then have to ask are, does any of this alteration affect the microbial activities occurring, and more importantly can this affect the safety of waste containment?
On one hand the excavation can increase microbial activity in certain places if cracks and voids remain between engineering materials and the host rock allowing the flow of groundwater and nutrients. On the other hand, elements of the design of the engineered barrier put in place create conditions that limit microbial activity, such as high pH (from cements), limited pore space and water availability (within highly compacted bentonite materials used to create a low-permeability barrier) or the waste itself could generate temperatures that will make it hard for microorganisms to survive. One challenge for us is to create these conditions in laboratory experiments and understand the circumstances under which microbial activity could affect the containment of waste. For example, could the activity of sulphate reducers affect the corrosion of waste containers and other metals in the repository? Could microorganisms consume gases such as hydrogen produced when metals corrode, and does this result in further corrosion-producing activity or does it reduce the pressure created by gas production? What about the role that microorganisms play in breaking down organic wastes and how does microbial activity affect the flow of fluids and the transport of radionuclides?
Similar sorts of questions apply to other industries such as those involved in the storage of waste carbon dioxide, storage of energy gases such as hydrogen and certain types of geothermal energy production and heat storage. Interest is currently very high within the hydrogen storage industry as people recognise that many microorganisms can obtain energy from hydrogen and their activity could lead to the loss of the stored gas, production of contaminating gases such as methane or hydrogen sulphide, clogging of flow paths by biomass production or mineral precipitation as well as the potential for migration of toxic hydrogen sulphide to the surface.
There are several microbial groups that are on the radar of storage operators. Top of the list are the sulphate reducers which are of particular concern because of the effects of the sulphides they produce. Historically, these organisms have been of concern within the hydrocarbon industry because they can “sour” gas reservoirs. They can also cause corrosion and precipitate minerals that reduce fluid flow, which combined with the potential to consume the stored hydrogen makes them organisms of interest in hydrogen storage. Our laboratory has observed that even low concentrations of hydrogen can stimulate sulphide production. They are not the only organisms that can consume the hydrogen in the subsurface, and the potential for methanogens (combined with the activity of acetogens) to convert the stored gas to methane is another area we are looking into. Of course, none of these microbial groups acts alone and so we are also interested in the microbial ecology of the whole microbial community in these environments. The use of high throughput sequencing techniques to do this is sometimes criticised (by the unenlightened) as stamp collecting but it can, on occasions, reveal the unexpected.
On a recent project we were conducting a preliminary survey of the microbial communities in groundwater around an open-loop ground source heat pump to see if its operation changed the microbial communities present. Our data showed that the microbial community had a very high proportion of methylotrophs. Subsequent dissolved gas analysis showed that we had discovered a site with exceptionally high methane concentrations which has opened up new possibilities for research on the source and cycling of this methane, its removal by microorganisms and potential impacts on the ground source heat pump.
Working on underground microbiology throws up several challenges. As if the low levels of microbial activity in some of these environments wasn’t challenge enough, the geomicrobiologist is faced with the very real problem of obtaining suitable samples and inoculum from hundreds of metres beneath the surface. This is a costly business requiring drilling operations, and usually the co-operation of industry in obtaining samples. Sample collection is further complicated by the fact that for microbiology, rock or fluid samples need to be handled and preserved quickly and cleanly to limit contamination and changes to the community after samples come out of the ground. Recently, we have been part of the team working on sampling rock core from the UK Geoenergy Observatory in Cheshire (and previously from the Glasgow Geoenergy Observatory). The Cheshire site is designed to test the effects of heating and cooling e.g. for heat pumps or heat storage and the Glasgow site focusses on geothermal heat from abandoned mine workings. At the Cheshire site, a sampling laboratory in a shipping container was set up which allowed us to sub-sample cores as they came out of the ground, record them and preserve them for future microbiological analysis. A set of samples from both these projects has been stored at -80°C, and access to them, along with associated data can be applied for from the UKGEOS project.
An additional challenge is that if we want to study microbial processes in the laboratory, we need to recreate the temperatures and pressures seen in the subsurface. Here we work alongside other BGS laboratories who have a wealth of experience of reproducing these conditions in the lab. We have devised a range of experimental apparatus that allow us to run complex experiments integrating biological, chemical and physical processes and monitor microbial activity under realistic conditions.
The variety of topics we work on keeps things fresh in the laboratory, and as if the range of industries mentioned so far isn’t variety enough, the laboratory also investigates the recovery of metals and rare earth elements from ores, mining waste and electronic waste by microorganisms, geotechnical applications of microbially induced carbonate precipitation, bioremediation and the leakage of gases such as methane through soils. Though working in such a diverse field has many challenges it is good to know that our work could play a small part in enabling a greener decarbonised future.
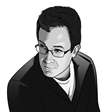
No comments yet