In September 2000, building upon a decade of major United Nations conferences and summits, world leaders came together at United Nations Headquarters in New York to adopt the U.N. Millennium Declaration, committing their nations to a new global partnership to reduce extreme poverty and setting out time-bound targets - with a deadline of 2015.
This became known as the Millennium Development Goals (MDGs). The eight goals focused primarily on improving various conditions in impoverished countries, mainly in Africa, East Asia, and South America. While many targets were met, a few remain unmet. Post-2015, the 17 Sustainable Development Goals (SDGs) build on decades of work by countries and the United Nations, including Agenda 21 of the 1992 Earth Summit and Millennium Summit of 2000. At the heart of the SDGs is an urgent call for action and global partnership by all countries – this time both developed and developing. Decades-long lessons recognised that ending poverty and other deprivations must go hand-in-hand with comprehensive strategies that improve health and education, reduce inequality, stimulate economic growth, protect life in water and on land, and tackle climate change.
Sustainable Microbiology
Our world’s challenges are diverse but appear well captured within the SDGs. But then, how can research in microbiology laboratories support feeding nearly 10 billion people by 2050 in a way that improves economic development while reducing pressure on the environment? How much can microbiology contribute to a sustainable future? Can microbes save the planet? Answering these questions needs to be built from and on the bedrocks of some fundamental truths – the certainty that microbes know no borders and are skilful at inhabiting, adapting, surviving, and thriving in extreme and constantly changing environments. Microbiology is directly linked to achieving SDGs addressing food security, health and wellbeing, sanitation, and clean water. These were problems that have been identified for many decades, and many practices, programs and policies are in place to address them. However, there are new entrants to the list of issues we all face. There is a vast entreat for strategies to increase affordable clean energy, build sustainable cities and communities, reduce economic inequalities, stimulate responsible consumption and production, and tackle climate change. These new globally acknowledged areas are non-classical, ambitious, yet attainable goals. Applying microbes and their products may be a sustainable pathway to solving these issues, though not necessarily having all the boxes ticked by 2030. At the heart of sustainable microbiology is the growing field of microbiomics.
Microbiomics is the science of collectively characterising and quantifying molecules responsible for the structures, functions, and dynamics of a community of microbes living together in a given habitat. Already research is moving closer to harnessing microbiome information to improve health outcomes. The idea of ‘community work’ of microbes in a complex system looks promising in producing microbial-influenced affordable clean energy. Furthermore, in the past, environmental research and policy have centred on natural or urban outdoor environments. For the past ten years, we have been developing an understanding about the complex microbial ecosystems found in the built environment.
In a few decades, microbiologists, or shall I say ‘microbiomists’, will be conspicuously seen alongside architects, surveyors and other construction stakeholders in the planning and designing of buildings and structures. Microbiology stands to become a key partner in the built environment when microbiomics is deployed and will significantly contribute to the safety and resilience of cities and communities.
Affordable clean energy (SDG 7)
About three billion people still depend on unsustainable sources such as wood, coal, and charcoal for cooking or heating. Energy remains a dominant contributor to climate change. Nothing changes in climate change if energy sources are fossil-based, unsustainable, and not green. Increasing the share of renewable energy in the global mix is one of the targets that appeal to microbiology the most. The action of microbes on domestic and industrial wastes, plants and agricultural substrates is increasingly being employed in the production of biofuels. Lignocelluloses are everywhere – wheat straw, corn husks, grass, and rice hulls. It is no news that biotech companies are racing to optimise the technology and microbes to produce efficient, affordable, and clean energy from lignocellulose sources. Several years ago, I had the thought that since a typical vegetarian diet contains plants with lignified cell walls, examining the gut microbiota and microbiome of people on high fibre may help elucidate lignocellulose degradation and perhaps reveal novel microbial enzymes. This background was the focus of my PhD, which used culturomics and microbiomics to search for microbes and enzymes that will depolymerise lignocellulose to accessible substrates. My findings were that for complete lignin-hemicellulose degradation, a consortium of cellulases and other enzymes from various bacteria has to be employed. Specialist bacteria such as Prevotella copri, Faecalibacterium prausnitzii, and certain Bacteroides species in consortia must be introduced to cover all the degradation steps and pathways.
Microbial-based biofuels hold promise to replace fossil fuels. For instance, Exoelectrogens – microorganisms that can transfer electrons extracellularly, are being researched in developing microbial fuel cells (MFCs). This bio-electrochemical system holds potential in the production of sustainable energy. MFC borders around employing microbial catabolic activity directly to generate electricity from degraded organic matter – conversion of chemical energy into electrical energy with catalytic reaction from microorganisms. A study in Indonesia by Juliastuti and colleagues utilised Escherichia coli and Shewanella oneidensis on wastewater from a sugar factory for a microbial fuel cell. Co-culturing of the two bacteria yielded higher voltage than a single microbe. An effort like this could potentially knock down the cost of wastewater treatment and recycling to generate electricity. There is increasing research in this area, and is covered in a review by Santoro and colleagues.
Recently, the low output MFC voltage (ca. 0.4 V) was successfully boosted to 2 V. That looks little and not enough. Yes, it’s not enough for our requirements, but it’s progress. Of course, most phones and other devices’ output voltage and amperage are 5V/2.4A. For fast charging, manufacturers bump the voltage from 5V to 9V or even 12V and/or increase the amperage to three. The low-energy output with MFCs from a single microbe calls for using a community of microbes to generate higher voltage output. Combining microbiomics will allow checking how bacteria, for example, in wastewater, interact as a community in the conversion of chemical energy to electrical energy and how specific species interact with themselves within the microbiologically large population. Perhaps microbes could be more organised in a way that bumps up the voltage, nearing our electrical requirements. Similarly, algae, a source of third-generation biofuels that produce several low-volume of, high-value products, has been suggested to integrate with bioelectrochemical systems like MFC. for sustainable and efficient bioelectricity production.
Aside from the proposal for an integrated third-generation biofuel, the advancements in genomic technologies have increased efforts on fourth-generation biofuels – energy derived from a combination of genomically prepared microorganisms and genetically engineered feedstock. Fourth-generation biofuel production incorporates carbon capture and storage (CCS) technology to create a carbon-negative, hoping to mitigate climate change as it would be expected to exceed all carbon-neutral options currently available. An increasing partnership exists between microbial researchers and energy/petrochemical companies for modified microbes that produce fuels on demand. Microbes are being modified to produce fuel nearly identical to diesel in the bioconversion of waste vegetable oil from fast foods. The main obstacles to the large-scale industrial development of microbial biodiesel include high production cost, enzyme stability and reusability. One of the most studied electrogens is the Shewanella strains, which are currently being explored in bioelectricity, including powering a future NASA space mission. High throughput sequencing allows researchers to study the effects of the space environment on this organism.
Sustainable cities and communities (SDG 11)
The world’s population is increasing, and such growth needs to be matched to accommodate everyone. Our survival and prosperity will require new, intelligent urban planning that creates safe, affordable, resilient cities and communities. The microbiome of built environment (MoBE), though a relatively new sub-discipline, has the potential to make contributions to safety, resilience and survival that were enshrined within SDG 11. MoBE studies the communities of microorganisms found in man-constructed environments – buildings (such as homes, offices, hospitals, classrooms, subway stations, libraries, and farmhouses), vehicles (such as aeroplanes, trains, and space vehicles), and water systems (such as shower heads, children’s paddling pools, plumbing systems). The microbiome of cultural heritage items, kitchen sponges, and household appliances has also been examined. Microbes are invisible inhabitants that are part of and affected by our environment. Within this discipline, there are opportunities to design a built environment with probiotics and other beneficial microbes to support the health and well-being of the occupants.
Some studies show that occupants living in the same building over time will have similar skin microbiomes. In another instance, children who grow up on farms have lower rates of asthma than the general, non-farm population, as specific groups of bacteria may have strategies that work against the development of asthma. In another instance Bacillus sphaericus spores enable self-healing of cracks, eliminating the need for traditional damage monitoring and repair and ultimately reducing maintenance costs. Equally, bacterial microcapsules create a green concrete wall. ‘Bio-informed’ design is getting the attention of architects and other structural professionals, and scientists can make this merging field relevant and applicable to address health safety, building resilience and sustainability to include reduction in bio-deterioration of building materials. Microbiomics concepts, tools and potential applications are expanding and are essential toolkits in deploying sustainable microbiology relevant to the global goals.
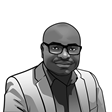
No comments yet