Included in this article is a link to the Kickstarter campaign to bring a graphic novel about the life of Fanny Angelina Hesse to life. If you enjoy the article, please consider donating.
Many microbiologists don’t know the secrets and wonders that the transparent substance they use every day still conceals.
In March 2023, a post on the platform then still called Twitter caught my attention. In a short thread, Ákos T. Kovács explained why it took his team “9 years of work and 20 authors” to complete a study they had just preprinted on bioRxiv. The reason why the post still holds my attention over a year later, I believe, is because it carries many reasons why I love microbiology—along with the realisation that there is an invisible force affecting our lives which, no matter how hard we dissect, control, and magnify, never ceases to amaze.
The playground for this amazement—those for the invisible realm of microbes—is agar. But for an increasing number of studies, including Kovács’, agar is a bottleneck both unparalleled and unshakeable. Agar conceals much more than what it shows on the surface.
“Alone they are practically irrelevant”
The study by Kovács and his team is one of the growing number of studies on mixed cultures (see graph), a way of bringing together two or more species in a controlled setting and seeing what happens. The researchers (who had just moved from DTU Denmark to Leiden University, The Netherlands) and their collaborators had observed previously, that two microbes often studied in the lab (the bacterium Bacillus subtilis and the fungus Aspergillus niger) interact with unexpected results: the bacterium attaches to and proliferates on the fungal hyphae thanks to matrix proteins, and both partners alter their metabolism in response to each other.
But what the team was searching for was an understanding on how these two species adapt to each other in the long-term, for example when they naturally interact in soil. So they grabbed an agar plate and developed a method to repeatedly grow the bacterium on fungal hyphae, and looked at what happened. By forcing the bacterium to evolve in such a way, the team identified a molecule it secretes (the lipoprotein surfactin), its target (on the cell wall of the fungus), and its mode of action (bulbous-shaped hyphae).
Importantly, the team found out that when B. subtilis produces more surfactin, it can inhibit the growth of other Aspergillus fungi, including pathogenic ones. Taking so many years for a study, now published in Nature Communications, might seem obsessive, but Kovács doesn’t agree:
“We had many observations from many experiments, but we couldn’t yet fit all the bits together. As we didn’t have specific funding for this project, we were free to go into various directions. I’d rather say that our study was an exploration into all aspects of the phenomenon we observed”.
What stands out from the study is how difficult experiments involving different species (called mixed cultures, or co-cultivations) can be, even when they strongly reduce the complexity of real-life microbial interactions. Think, for example, at the astonishing complexity of any microbiome, or that just two microbes are rarely alone in nature. Even if so, a scenario that allows for one interacting partner to remain the same over time is unlikely.
These experiments are cumbersome because agar plates must accommodate different species (with their, often very different, nutritional and environmental needs); thus, mixed culture experiments with three or more species are very rare. Mixed culture experiments without agar are possible but cannot accomplish what solid media does. Liquid media, for example, are not well suited to studying cells’ points of contact, or to easily separate the mixed cells for independent analysis and repeated culturing, like in the experiment led by Kovács.
Lucia Muggia at the University of Trieste agrees that one of the reasons why lichenology is an understudied discipline is because it is so difficult to grow lichens and their symbiotic partners on agar plates. “A hot topic in lichenology at the moment is finding the determining factors that make two partners form a symbiosis”, she explains. She pauses for a moment, before adding: “Alone they are practically irrelevant”.
Muggia and Kovács are in good company in their struggle to grow mixed cultures. The past decades saw an incredible rise in mixed culture experiments, as shown in the graph above. Scientists do them for a whole lot of reasons: to have a mechanistic understanding of microbial ecology, optimize industrial processes, discover new substances, and grow new species.
Mixed-culture experiments allow researchers to have control over incredibly complex settings. And while it’s ironic that a breakthrough in modern medicine happened thanks to a serendipitous contamination (when Alexander Fleming discovered the fungus producing the penicillin antibiotic), purity allows for understanding impurities; control allows us to harness the power of serendipity. Microbiology (and, probably, every discipline in science) is about having tools to make sense of the world.
Agar is indeed such a good tool for microbiologists that it has become irreplaceable: none of these 10,000+ co-cultivation studies are done without agar, in one step or another. When one cannot do without a constraint, isn’t that a curse? To answer this question, it’s worth looking back at when it all started, at a time when agar wasn’t as good as coagulated blood.
Seeing is not believing
Contrary to what many microbiologists think, the introduction of agar in bacteriology (and from there, in the whole life sciences along with its derivative, agarose) hasn’t been a watershed moment, but rather a slow avalanche that has taken over–everything, yes, but after some time. Thus said Robert Koch (1843-1910) with the first mention of agar in the scientific literature:
“On this medium the bacilli form only irregular small crumbs, which are not nearly so characteristic as the growths on blood serum”.
Robert Koch didn’t yet realize the importance of agar.
The “bacilli” he mentions are tubercle, or Mycobacterium tuberculosis. In a true tour de force, Koch isolated for the first time the bacterium causing tuberculosis. The achievement granted him immediate, worldwide fame and was hailed by colleagues like Friedrich Löffler (1852-1915) as “a world-shaking event”. Koch showed remarkable technical prowess. Mycobacteria are slow growing, as any microbiologist who prefers working with E. coli will tell you, and their thick, lipid-rich cell wall make staining, and hence microscopy, difficult.
But that’s not the only reason for Koch’s accolades: with rudimentary laboratory equipment, a young Koch proved for the first time the parasitic nature of a human infectious disease – and one that killed up to 1 in 7 people in Germany at the time. Koch’s discovery led him to formulate his “Koch’s Postulates”, which establish causation between a pathogen and its infectious disease, and to win a Nobel Prize in 1905. In the meantime, a quiet revolution was slowly incubating.
Cloth merchant and self-taught scientist and lens-maker Antoni van Leeuwenhoek (1632-1723) had first seen microbes over 200 years before Robert Koch’s work. But seeing microbes wasn’t enough to make a physician believe they cause diseases: many argued that microbes were just present at the site of infection. Lenses and microscopes magnified cells into images, but solid growth media magnified cells into colonies—and thus into something researchers could work with.
As Georg Gaffky (1850-1918) said in his 1910 eulogy to Koch:
“It was only now possible to isolate the individual species from a mixture of bacteria with certainty, to maintain them in pure culture for as long as desired and to carry out precise experiments with them. […] To use Koch’s own words, after the right methods had been found, the discoveries fell into his and his colleagues’ laps like ripe fruit”.
The “right methods” involved, of course, agar and Petri dishes. While Julius Petri published a short report in 1887 that immortalised his name with namesake dishes, the revolution grown from agar made less of a stir. In fact, many microbiologists would struggle to mention the person who first suggested using agar instead of gelatine (or blood serum). It wasn’t Koch.
Unknown history
Fanny Angelina Hesse (1850-1934) is the woman behind the introduction of agar into microbiology, but few people know her name. Married to Walther – a bacteriologist who spent a research stay in Robert Koch’s lab in Berlin in the Winter of 1880-81 (what would be a Postdoc today) – the role that her time and place assigned to her was that of a “German Hausfrau”. Besides household chores (and the demanding job of raising three kids), she documented and preserved the work of her husband (the cover image for this article is one of hers).
Perhaps it is human nature to take for granted something which we can’t do without. Perhaps for this reason microbiologists don’t think often about the origin of agar, except in moments of need. Recent shortages of seaweed (from which agar is isolated) amidst over-harvesting on the coast of Morocco might have been a wake-up call. During WWII, the British government certainly realised the importance of agar when it issued a national emergency following import shortages from Japan (the major producer at the time), and started a UK-wide foraging across its coasts. But none of this helped in bringing any posthumous recognition to the mind behind the use of agar in the life sciences.
Fanny Angelina Hesse’s story is fascinating, and after a first historical account a few years after her death, the only other source about her comes directly from her grandson Wolfgang. Because of her Dutch heritage, Angelina knew that people in colonised Java (present-day Indonesia) used agar in puddings instead of gelatine. The story goes that the couple tried to grow microbes on agar, saw that it isn’t easily molten by heat and microbial digestive enzymes (as is the case for gelatine), and tested its superiority as a gelling agent. They then sent a letter to Koch to communicate this.
The rest is history. Well, kind of. Koch never acknowledged the Hesses for this insight, but it’s hardly a case of scientific misconduct as the importance of agar eluded him. The fact that until now we have had scant information about Angelina and Walther Hesse certainly contributed to their consignment to oblivion.
A side note for the history buffs: the term “colony” when used to refer to the growth of microbes is, perhaps, the only one without negative connotations. And yet Koch’s first use of the term could be ascribed to geopolitical tensions between the colonial states of France and Prussia. Interpersonal conflicts between Koch and Louis Pasteur, caused by stubbornness and an impasse with an interpreter during an international congress, likely played a role too. It’s safe to assume that our modern scientific culture keeps us safe from those interpersonal conflicts, if perhaps only of the latter cause.
The world on an agar plate
Growing microbes as pure cultures free from contamination has been—and still is—fundamental for microbiology. So much so that the “Koch’s plating technique” (involving the streaking of cells on a semi-solid agar surface in Petri dishes) has basically remained, invariably, for the past 140 or so years.
But, as the name implies, pure cultures are antithetical to mixed cultures. The issue with purity is that it doesn’t help in understanding how microbes interact, and microbes are never alone in nature (except perhaps in extreme environments or in host tissues). But if agar is a constraint, how to replace an essential technique? Is the issue perhaps in the setting—should microbiologists stop trying to recreate the microbial world on an agar plate? Or is the limitation within our scientist selves—could A.I. and automation help?
Researchers know that ecological context matters, as well as for microbes. Context can, for example, explain the limitations of “Koch’s postulates”. Some pathogens cause disease in some people and not in others, for example, a commensal (a species living with a host without causing much benefit or harm) can wreak havoc only in certain circumstances. To better understand this complexity, some researchers apply “ecological Koch’s Postulates”, which are akin to “Koch’s Postulates” but consider the ecological context. Other say that, if this ecological context is the human body, the term pathogen should be ditched altogether.
Much of this complexity comes from the difficulty of dissecting cell-to-cell interactions, which might explain why many findings about the microbiome are correlational, rather than causal. A recent study finding fungal cells associated with cancer left scientists with the question of whether their presence was a cause of cancer or the aftermath, in a striking resemblance to pre-Koch debates about the nature of infectious diseases. A suggestion for microbiologists studying complex microbiomes is to develop “model communities” akin to “model microorganisms”.
And perhaps an agar plate isn’t the best ecological context, anyways. About 150 years after Koch, about 350 years after van Leeuwenhoek, scientists still struggle with being able to see microbes. Matthias Rillig at the Freie Universität Berlin is slightly irritated that he cannot see the “true, inherent shape” of fungi despite studying them for decades. “Agar plates only create a two-dimensional approximation.” The thought occupies his mind so frequently that he offers any undergraduate student coming up with a realistic project proposal the chance to do a fully funded PhD in his lab. “If you study birds, you’d want to see what a bird looks like.”
Molecular methods like DNA sequencing have revealed secrets concealed by agar. One of them is the “great plate count anomaly” that scientists observed pretty much as soon as they started plating; that is, the fact that they can see more cells under a microscope than colonies on agar after plating that same sample. The sheer size of this anomaly (only an estimated 1% of observed cells grow on agar) points towards the assumption that 99% of cells aren’t dead or dormant, but rather non-culturable. To know what these 99% of cells are, and (potentially) do, molecular methods help.
But then again, microbiologists do more than observe cells and analyse DNA, and thus they need colonies. To culture the “unculturable”, some scientists grow samples in their natural environment while maintaining purity with semi-permeable membranes. Those membranes allow a chemical exchange with the environment but prevent an exchange of cells and thus contamination. The setting creates compartments without the pitfalls of agar: the natural environment could provide nutrients unavailable in agar plates, for example, or the guarantee that toxic by-products of agar aren’t present. These form during autoclaving agar with phosphate and are one of the reasons for the “great plate count anomaly”. And while alternative gelling agents (for example, gellan gum) might help in growing the “unculturable”, they maintain the setting, and pitfalls, of purity.
Researchers can achieve scalability by reducing the size of the compartments. The “isolation chip” (or “iChip”), for example, is about 7cm by 2cm in size and has 192 compartments (as a comparison, a Petri dish has a diameter of 9cm, and one compartment). With this and other tools, microbiologists can attempt to grow the so-called “microbial dark matter” (the “unculturable” 99%), an achievement often pivoting on the fact that many species need other species, or products of their metabolism, to grow. In other cases, incorporating microbial interactions has led to the discovery of new metabolic products, including antibiotics.
Many more devices and approaches for miniaturisation exist. But increased sophistication doesn’t make these tools more accessible to scientists, often already on tight budgets and thinly stretched. Also, most tools (including the “iChip”) still use agar, and “domestication” – that is, growing wild species, often repeatedly, until they easily form colonies – is done on agar plates, too. Even when they venture into the field, microbiologists need to return regularly to the lab bench.
If field experiments are excursions in between irreplaceable bench work, and if new, smaller tools still can’t do without agar, what then can automation and AI do to help? As of now, these technologies hold great potential and are yet underdeveloped. Researchers have used them to let the machine perform autonomous experiments, or to make sense of complex microbial interactions in the environment. Perhaps one day microbiologists will be able to integrate various tools for automated, miniaturised, large-scale experiments without agar. If this future will first replace the hands of the experimenters, or the substratum of the microbes, is hard to tell.
It goes without saying that microbiologists should, despite the hard work they are already putting in, keep investigating the microbial world, one agar plate at a time.
Making the invisible visible
It’s ironic that something as transparent and ubiquitous as agar conceals so much. Agar hides secrets about microbial interactions perhaps because it isn’t the best substratum to reveal them. But agar is irreplaceable and will probably always be used in labs, if “only” at the beginning (to streak, isolate, and grow cells) and end of experiments (again to isolate, and then preserve strains).
Whatever the substratum (agar, or another gelling agent), tool (a Petri dish, or a miniaturised device), or intelligence (human, or artificial), another invisible force is just one co-cultivation away: microbes’ metabolism. Even well-studied species have many inactive (a.k.a. silent) genes whose unknown products are difficult to determine in a laboratory setting. This “secondary metabolism” is not involved in primary functions (like growth, cell division, and the like) but can nonetheless have life-saving effects – for example when microbes produce antibiotics to outcompete other microbes. In our future plagued by the threat of antimicrobial resistance, microbes’ secondary metabolism will have life-saving effects for us, too.
And then there is, sadly, the invisible contribution of Fanny Angelina Hesse to the life sciences. Voices on the internet are growing, calling for Angelina’s story to be remembered. Together with a group of artists and science communicators, we want to do exactly that, bringing her story – and that of agar – to a broad audience with a graphic novel called “The Dessert that Changed the World & the Forgotten Story of Fanny Angelina Hesse”. Our story will be based on new historical source material about Fanny Angelina Hesse obtained from surviving relatives (her great-grandchildren), which includes original scientific illustrations, unseen family portraits, and an unpublished biography written by grandson Wolfgang Hesse. Please support us in our Kickstarter campaign, or by helping to share the news, if you can!
When something important is ignored, wonderment can become annoyance. When hard work can’t solve mysteries, dedication can seem like obsession. We watch microbes and the Petri dish become a ring, with agar as the battleground. We witness a competition between control and serendipity, purity and contamination, artifice and nature – and, in less naturalistic terms, the clash between a culture of remembrance and the inertia of oblivion. Whatever side one chooses, it’s a fight worth watching. Agar still has a few secrets and wonders to reveal.
Dr. Corrado Nai spent a lot of time using agar, and did a postdoc on microbial co-cultivations with scientist and artist Vera Meyer in Berlin. He is currently full-time parenting while writing a graphic novel about the story of agar and Fanny Angelina Hesse.
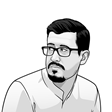
No comments yet